One photon...many pieces of information
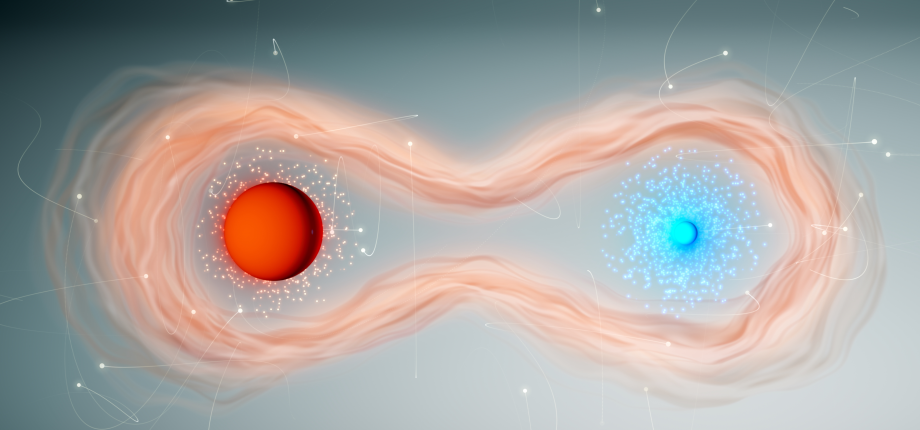
It's a well-known fact that at the sub-micrometer scale, where your work focuses, the physics at work differs from what is observed in the macroscopic world...
Yes, with quantum physics, we have to put aside classical physics and go beyond what our brains can traditionally imagine. The model that governs the interactions of the infinitely small is based on three major principles that may come as a surprise. The first, superposition, indicates that a particle exists in different states. Thus, a photon may be red and/or blue until it is observed. It is only when it is observed - and this is where the second principle of measurement effect comes into play - that the photon's state is determined. The particle “chooses” one color or another at random. In fact, there is no mathematical equation to describe and predict this choice, as Newton's laws would allow when flipping a coin. The final pillar of quantum physics is entanglement. Two particles are said to be entangled if the measurement of the state of one determines the state of the other, wherever they may be. Let's take the example of the red and/or blue photon and produce a pair of entangled photons in Paris. Let's send one to Berlin and the other to London, then observe them separately, without the possibility of communicating the results. According to the principle of entanglement, if one photon measures red, the other will measure blue (and vice versa). So, if the Berlin photon turns out to be red, the London photon will instantly be blue, without any exchange of energy or information.
All this takes us out of a binary system where light is either off or on, for example. Information is no longer encoded with bits at two levels of information - 0 or 1 - but with qubits offering more combinations, and therefore the possibility of encoding more information.
Your role is to investigate how these properties can be exploited to develop quantum technologies, in particular with single photons. How do you use them?
At the heart of my research is the use of frequency-intersecting photon pairs, produced using a specific optical process (interaction of an intense field in a non-linear crystal at room temperature). A single photon has a whole range of characteristics called degrees of freedom - frequency, polarization, or its distribution in time and space - which are useful for encoding quantum information. While photon polarization has so far been widely exploited in quantum communications protocols, my research group is exploring the potential offered by photon frequency (i.e. photon color over a certain frequency range). This degree of freedom is particularly robust, as it is less exposed to interactions with the environment. The idea is to make these single photons carry as much information as possible. In concrete terms, instead of considering a photon under a single frequency - that corresponding to red, for example - and associating a single piece of data with it, we use different colors/frequencies to encode different units of information on the same photon. Blue would be the logical 1, then yellow the logical 2, etc., following the spectrum of light up to red. We thus create an alphabet, called colored qudits, where the “d” refers to the number of colors in the encoding. A qudit then accepts more information per photon than a simple qubit.
Once you've established these possibilities, what are you going to use these unique photons for?
Metrology, for example, with the aim of increasing the precision of measurements of physical parameters (distances, magnetic fields, etc.). By playing on the color of the photons, but also on their entanglement, we are creating new types of highly sensitive probes (they can detect time shifts of the attosecond order, i.e. 10-18s) giving access to particularly high measurement resolution. All this without the need for intense optical fields, whose excessive energy would damage fragile objects such as biological samples.
My work is also aimed at the field of quantum communications. Thanks to frequency-interleaved qudits, a single photon carries more bits of information, making it possible to design a signal that is more resistant to the disturbances (noise) generated by its propagation in a network. Another advantage of using quantum resources is that any perturbation to one of these photons can be detected. In other words, any interception of one of them in the middle of a communication channel will be spotted and characterized precisely, regardless of the capabilities of the attacker's devices. This is an asset in terms of communications and network security, which has no equivalent with traditional resources. Finally, several users can share different frequency bands, and therefore different colors of entangled photon pairs, making it possible to design a quantum network.
About:
Nicolas Fabre is senior lecturer at Information Processing and Communications Laboratory (LTCI) at Telecom Paris, specialized in quantum information and quantum optics. He works in partnership with the Materials and Quantum Phenomena Laboratory (MPQ) laboratory of the CNRS and Université Paris-Cité, the Center of New Technologies in Warsaw (CeNT) and the Istituto di fotonica e Nanotecnologie in Rome.
>> Nicolas Fabre on Google Scholar
>> Nicolas Fabre personal webpage
*LTCI: a research lab Télécom Paris, Institut Polytechnique de Paris, 91120 Palaiseau, France